The Two Main Types of Fingerprint
All the rocks (mainly basalts) of the oceanic sea-floors generated along the oceanic spreading centres; in the oceanic islands (the OIBs) and those linear chains lying along fracture zones such as Tahiti, Hawaii, the Austral Islands, the Louisville Seamounts or the Azores etc all have a regular multi-element fingerprint, domed slightly in the middle when normalised to primative mantle or MORB composition. The basalts of the mid-oceanic rises may differ somewhat, tending to be very LILE depleted, that is, they slope down to the left as the Cs, Rb, Ba, Th, U, Nb, K, and light REE are present only at very low levels in common NMORBs but the basic shape i unchanged.
All rocks generated along subduction zones, where the oceanic crust underthrusts the continental margins, (mainly andesites-dacites, with rhyolites and intruded granodiorite-granite where the crust is thick) have a quite different finger print identified by low Ti, Nb, tantalum with high Pb, and relatively high K, U, Th, Ba and especially Cs. As we will see, the amount of the K element group present can vary by a factor of nearly 100 as we go from island arcs located at very thin crust, (~15km thick) to lavas generated in the middle of continents where the crust is 50km thick or more. All continental rocks have this fingerprint regardless of whether they were associated with an orogenic zone or not. eg the analytical standard W-1, the Centerville Diabase, which comes from a quarry near Washington DC on the way out east, has a typical continental signature, though in major elements it does not differ much from Kilauean basalts on Hawaii except in being slightly more potassic. Sediments including mudstones and even sandstones have the same signatures suggesting that virtually all continental rocks (with the exception of a few alkali basalts) originated as andesites, however weathered and or metamorphosed they may have become.
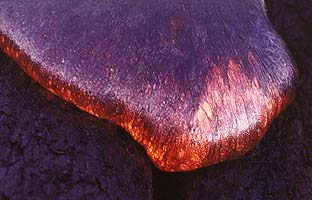
A lava toe, Kilauea (Photo: Glen Kaye)
 |
Typical Fingerprint of a lava derived from the sub-oceanic lithosphere. In this case, a slightly alkaline series from off Southern Iceland. |
 |
Fingerprint of calc-alkaline andesite from Lanin Volcano, S. Chile, and typical of any magma derived from the sub-continental lithosphere, with the exception given below. Pb regrettably not included but is symmetrical in oceanic rocks, enriched in continental rocks as is the Cs, Rb, Ba, U, Th, K. |
There is one oddity...
Alkali basalts, ie those with a high K, Na content cf silica, retain the same Oceanic Crust signature no matter whether erupted as Oceanic Islands, or along the continental margins (usually along fault zones) or even those quite rare ones which appear along faults in the middle of the continents, such as those along the Cameroon line or the East African Rift. Perhaps they come from too deep in the mantle to be affected by the composition of the overlying continental mass.
Fingerprints in Related Magma Series
As we said above virtually every volcano and even magma batch has at least slightly different chemistry to any other. This chemical pattern is called it's "Fingerprint" The most useful one is shown by the "Mantle-Normalised Diagram" by Sun & McDonnough showing the relative concentrations of 23 minor and trace elements, ie Cs, Rb Ba, Th, U, Nb, K, La, Ce, Pb, Pr, Sr, Pb, Pr, P, Nd, Zr, Sm, Eu, Ti, Dy, Y, Yb, Lu when "Normalised" to estimated "Primitive Mantle" composition. They have to be normalised to something, otherwise with amounts ranging from less than 0.5ppm to up to 10% they could not be shown on the same diagram. One soons gets ones eye in and says knowingly,
"aha! 40 to 60 times mantle! Pretty enriched, what?"
When Cs is at 0.5ppm, levels in one series but 3ppm in another, it may not sound much, but a six times enrichment is, very definitely, significant. Within a single related magma series from basalt to granophyre a total range of enrichment of perhap 100 may be seen for a few elements but in differentiated basalts derived from a single parent the range is is seldom greater than about 10.
The rules are:
- No two volcanoes seem to include flows with exactly the same fingerprint for all elements. Either the scale or the relative abundance of elements will be different.
- Some volcanoes, eg, Mt Shasta, show amazing differences between different parasitic cones, eg when compared to Shastina or Black Butte, and even between lava flows.
- The fingerprint will change as different minerals appear on the solidus. Either these minerals have been removed taking with them some high percentage of some element, or the magma has been buffered against the mineral during formation.
- In most basaltic magmas:
- The first mineral to form at temperatures above 1200degC, is an aluminous spinel. There are only tiny amounts of it, but it extracts most of the Cr+++ which will decline very rapidly.
- Olivine is usually next, (Mg, Fe, Ni, Co, Mn, Zn) SiO4 in magmas of 10% to 7% MgO. (But in ORBs usually accompanied by plagioclase). This whips out up to half the MgO, most of the Ni, (up to 3000ppm from a magma with only about 400ppm in it), and most of the Co, while Fe, Mn and Zn have a partition coefficent of about 1 and remain about the same no matter how much olivine is added or removed. Olivine cumulates are called "Picrites".
- At about 7% MgO, (especially in Hawaii) down comes clinopyroxene-plagioclase. Ca which occurs in both minerals, takes a dive, as does Sc which is heavily concentrated in Cpx, both of which were built up with Ol removal. Sr may decline or at least ceace to increase. Soda flattens out, Al (and Ga) goes on increasing slowly as the average Al2O3 in an Ol-Cpx-plag mixtures is less than the 14% in the magma.
- In an alkali basalt-basanite series at 6.5-7% MgO (or more), olivine and cpx form together in about equal amounts. The cumulative rocks are called "Ankaramites: They are later joined by plagioclase in smallish amount so the exact point is hard to define.
- At 4% MgO, down comes titanomagnetite. A big drop in TiO2, V, and Fe results.
- At 3% MgO, down comes apatite with a big drop in P, and some modification of REE. Neither of these last two minerals contain silica so at 3% MgO a marked increase in silica may take place especially in tholeiites but even in alkali basalts.
- At less than 1% MgO, an alkali feldspar (usually sanidine), comes out. This marked by a catastrophic decline in Ba and Sr.
- At about 0.1% MgO, out comes zircon with sharp decrease in Zr. Not many crystallising magmas go this far, so the Zr point is not often seen except in very fractionated rhyolites and in Craters of the Moon CFB commendites-trachy-rhyolites.
- This sequence is almost universal. No rock with only 3% of MgO even if it looks like a basalt, will have more than a few tens of ppm of Cr, Ni, Sc, Mg etc and Ca will be down to only 4-5%. No rock with only 2% MgO will have much Ti or P left, ie TiO2 might have gone from 4% down to 0.3%. So do not waste time looking in dacites or ryholites for Ti ores!
- Calc-alkaline or andesite series show a rather different sequence. I a range of rocks from 10% MgO to zero %, all the main elements Mg, Al, Ca, Fe, Ti, V, P decline at constant slope, while SiO2, Na2O, K2O, Ba, Rb, U, Th increase. There is rarely any break in slope suggesting a crystal phase has appeared on the solidus. There is no peak at near 4% MgO for Ti, V, P etc. Nor any changes in slope in CaO, FeO, Al2O3 etc. This suggests that crystal fractionation does not take place to any great degree in calc-alkaline magmas.
-
- If we look at the evolution of Hawaiian lavas using data from non-crystalline glasses only, we get some interesting results. Melson & O'Hearn analyzed several hundred glass samples as part of their Deep Sea study. Helz then analyzed the interstitial glasses recovered from drill holes in the 1959 Kilauea Lava Lake and these included samples of near rhyolite composition not found as rocks. Dave Clague who was resident geologist at Kilauea forwarded some unpublished data of glasses and unlike all the rest these include samples of more than 10% MgO. They may include re-dissolved olivine???
 |
CaO/MgO diagram. Between 6.5 and 7% MgO are a mass of points. This is the most common Kilauean magma. Melson & O'hearns data show an extension above 7% MgO towards, not olivine, but Ol+Cpx. At 7% MgO, the formation of Ol+Cpx+Plag together produces a sharp drop in CaO content. The Titanomagnetite Point at 4.5% Ti is passed without effect but there is a slight flexure in CaO at 3% MgO when Apatite forms. |
 |
Cpx contains some TiO2 so the trend flattens when Ol is joined by Cpx. The total MgO content of about 10% Ol, 40% Cpx and 50% Plag (the composition of this ternary eutectic) is about 9-9.5% and Ti increases steeply. At 3% MgO titanomagnetite forms and Ti diminishes rapidly. |
 |
Neither Ol nor Cpx nor Plag nor TiMt contain any P, so the P content increases steadily. At 2-2.5% MgO, apatite forms and the P content drops steeply. |
 |
Here the trend at first is towards increasing CaO and Ti. At the Ol+Cpx+Pl point the crystal cumulate contains about 16% CaO which drops but Ti continues in increase. At about 4.3% TiO2 titanomagnetite forms and Ti drops sharply. The Apatite point does not show any visible affect. |
- The most odd effect is seen in that there are three or four samples greatly enriched in Ti and P, yet these are glasses. Possibly titanomagnetites and apatites formed in the lava lake under the thickening crust but sank into hotter parts of the lake and were redissolved.
- There is rather broad scatter in the low Mg samples, but remember these are tiny interstitial fragments of glass analyzed with the electron microprobe and local effects can be expected. No rocks of this composition are found in Hawaii, but are elsewhere.
- All rocks show these trends. The Galapagos and Icelandic series show the TiMt point better as rocks near this composition are much more common, see section on "Oceanic Islands".
-
- The residual elements fingerprint diagram changes slope while this is going on. Cs which has the lowest partition coefficient may increace by a factor of 100 in a basalt-granophyre series, while Lu which has a partition coefficient of nearly 1 will stay about the same. The mantle-normalisation diagram has the residual elements arranged in order of decreasing bulk partition coefficient. This must be clearly understood or nothing will make sense.
- A somewhat similar shift will be seen as we go towards rocks which are more "alkaline" i.e. have more Na & K relative to silica. All tholeiitic rocks, ie those with excess silica, will have lower Cs, Rb,Ba than any alkali basalt. While there is a complete transition, I do not know of any alkali basalt with less Rb,Ba than any tholeiitic rock except in the oddly depleted Galapagos Is basalts
-
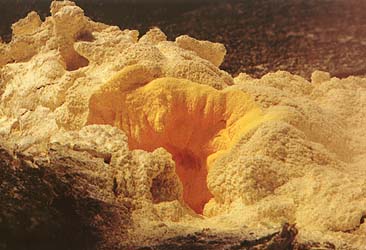
Another element separates.
Sulphur above a Puu O'o lava tube
- (Photo: Glen Kaye)
-
-
 |
Click here to see an intraplate alkali basalt series. The rocks are from the Puu O'o series which has erupted continuously since 1984, |
 |
The ABs from separate parasitic hawaiite cones on the extinct Mauna Kea.
Note the alkaline rocks are about 5 times more enriched. |
- Often while the Cs, Rb are much greater in the alkaline rocks, the Y, Yb,Lu may be lower. Therefore one can indicate how "alkaline" a rock is by giving the ratio of, say, Rb/Y or Cs/Lu (or any two elements at opposite ends of the normalised diagrams) thus putting a numeric value on the slope. Geochemists specialising in REE have long used a slope factor to indicate alkalinity. A La/Sm ratio of 1 is used to separate the N-Type, or depleted MORBS (Mid-Ocean-Ridge-Basalts) from the E-type (Enriched MORBS).
BACK
Copyright © 1998-2006 Dr B.M.Gunn
|